Update from our 2024 Future Materials Innovators Program students
Dec 6, 2024
2:30PM to 3:30PM
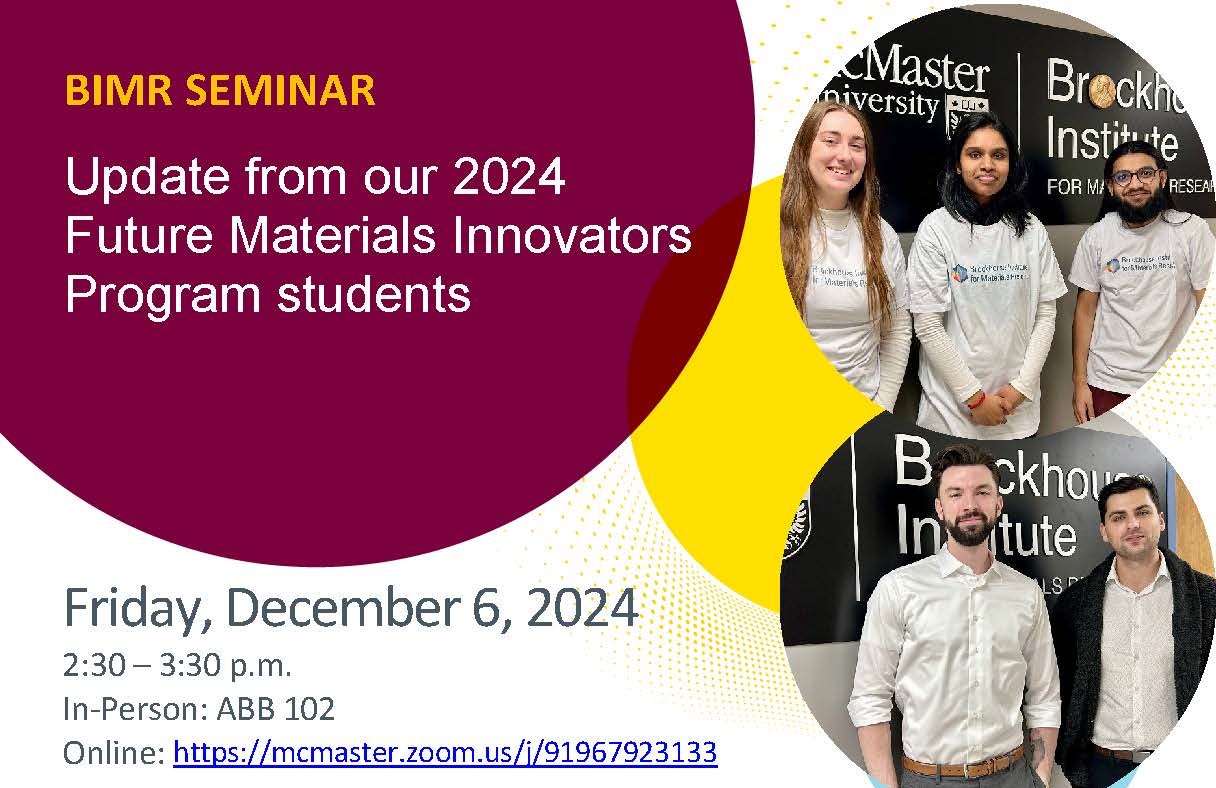
Date/Time
Date(s) - 06/12/2024
2:30 pm - 3:30 pm
Categories
1- Investigating the Impact of Shear Control on Battery Electrode Preparation at the Laboratory Scale, by Storm Gourley (Higgins Lab) and Nathan Mullins (Latulippe and De Lannoy Labs)
Coating and drying of electrode slurries is a critical part of manufacturing commercial scale battery electrodes, with all downstream production steps (e.g., calendaring, slitting/notching, winding, etc.,) depending on the quality of the electrode cast. While modern operations utilize roll-to-roll coating methods to minimize processing time and ensure inter-batch consistency, these techniques are often not feasible for laboratory scale research and development of battery electrodes owing to the high cost and large footprint of required infrastructure. As a result, laboratory scale electrode preparation is typically done using a manual blade coating method which offers minimal control over quality affecting parameters including the speed and height of the blade, introducing potential inconsistencies in electrode-to-electrode quality. This issue of variability is exaggerated by the lack of statistical rigor in battery research and development; whereby electrochemical performance for new cathode materials is often reported from the data of even a single coin-cell. This mischaracterization can lead to issues with performance validation when developing new materials, slowing the development of next-generation batteries. Herein, we use a novel, low-cost, automated casting system developed from 3D printer architecture to demonstrate the importance of shear control when preparing battery electrodes at the laboratory scale to obtain consistent replication of mass loading, thickness, morphology, and electrochemical performance. By accurately modulating the speed and height of the blade (± 7 µm) during the casting process, the variance in mass loading across a 150 cm2 electrode sheet was shown to be greatly reduced. Inter-electrode coefficient of variance was minimized to <7% (mass loading) when casting at a speed of 5 cm s-1 with a blade height of 150 ?m in comparison to 15% when casting is done manually. Taken together this work highlights the importance of holding a high level of control over electrode casting for lab scale research and development of next-generation battery materials.
2- Forming Oligonucleotide-Based Complex Coacervates for Encapsulation and Intracellular Delivery of Functional Proteins, by Annina Ashok (Bujold Lab), Kaitlin Davies (Bujold Lab) and Marzuk Gazi (Dalnoki-Veress Lab)
Proteins have characteristic function, and morphology that contribute towards wide variety of cellular function , making them an ideal candidate for potential therapeutic applications. However, proteins are limited by their inability to cross the cell membrane due to their inherent structural instability, surface charge and large morphology. To overcome this challenge, there have been advances in figuring out rational designs such as covalent-based modifications, mechanical delivery systems and non-covalent based carrier systems to facilitate functional protein delivery. Specifically, non-covalent based carrier systems are of interest, as spontaneous self-assembled structures do not disrupt the native protein structure and function. Here, we propose attempting complex coacervation with cationic and anionic oligonucleotides, with the future goal of encapsulating proteins. Complex coacervation is a process of liquid-liquid phase separation phenomenon that is caused by the electrostatic attraction between oppositely charged polyelectrolytes in solution. Previous studies have showed that complex coacervation is achievable for polymers and polypeptides. Based on previous literature, we have characterized coacervate formation using cationic poly-lysine, and anionic, single-stranded DNA. Using optical microscopy, coacervation of these biopolymers can be observed over long periods of time. By tracking the positions of the coacervates over time, we measure their diffusion coefficients and can use this to find the size. The ideal total coacervate concentration, which is the concentration at which coacervation occurs between two shorter oppositely charged polyelectrolytes, has also been determined to inform future coacervation attempts with oppositely charged oligonucleotides. The next phase of this project would be to attempt complex coacervation with cationic and anionic nucleic acids. Although there are many approaches for synthesis of cationic oligonucleotides, a standard solid-phase H-phosphonate based coupling cycle can be manipulated to attach an amine to the phosphodiester phosphate during the oxidation step of the coupling cycle. Although synthesis of cationic nucleic acids is possible, it is generally very challenging to achieve synthesis in high yields, or to synthesize the length of oligomers needed to achieve complex coacervation. With the aim of improving the synthesis and work-up process, we aim to functionalize the glass surface of standard DNA synthesis resin with 3-APTES. This is done to achieve a cationic surface on the resin to promote the dissociation of cationic oligonucleotides from the surface of the resin during synthesis and work-up. This attempt to improve solid-phase synthesis of cationic oligonucleotides will lend itself to the future synthesis of cationic DNA needed for complex coacervation with standard anionic DNA.
Followed by a BIMR Reception!
We are pleased to invite all our members to a Reception after the Future Materials Innovators Seminar, to celebrate the end of the Fall term. Join us to share a few appetizers and mingle with your colleagues.
Reception to follow the Seminar just outside room ABB 102.
In-Person: ABB 102
Online: https://mcmaster.zoom.us/j/91967923133
Meeting ID: 919 6792 3133
Passcode: 329366